Understanding Hydrogen Fuel Cells: Processes and Products
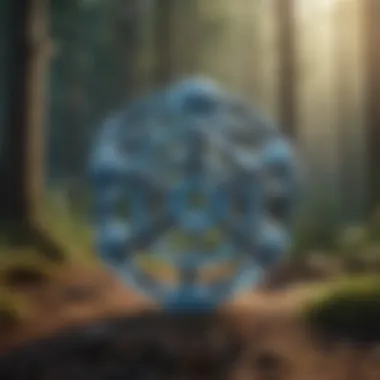
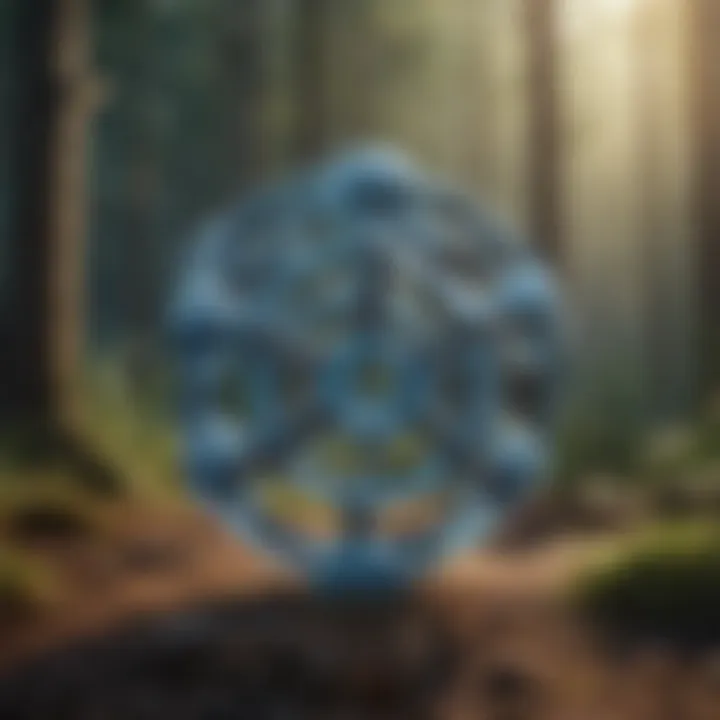
Intro
Hydrogen fuel cells represent a significant innovation in energy systems. They leverage hydrogen's chemical properties to produce electricity efficiently. Unlike traditional combustion engines, fuel cells convert chemical energy directly into electrical energy, yielding water as the primary byproduct. This process maintains high energy efficiency and emits fewer greenhouse gases than fossil fuels, underscoring its potential role in sustainable energy solutions.
In this article, we will examine the mechanics behind hydrogen fuel cells. Our exploration will focus on the combustion processes associated with hydrogen and the resulting output products. Furthermore, we will address how hydrogen interacts at the molecular level during energy conversion, the principles of electrochemical reactions, and the implications of these technologies for energy production and environmental sustainability.
Understanding these complex interactions is crucial for professionals in the energy sector, academics studying sustainable technologies, and policymakers aiming to promote environmentally friendly practices.
Preamble
The exploration of hydrogen fuel cells is critical for understanding the future of clean energy technologies. This article seeks to provide an in-depth analysis of hydrogen fuel cells, focusing particularly on their combustion processes and any resulting output products.
Hydrogen fuel cells operate on the principles of both chemistry and physics. The interaction of hydrogen gas, whether combusted or utilized within fuel cells, illustrates various energy generation methods. The benefits are substantial, including a reduction in greenhouse gas emissions and low environmental impact compared to traditional fossil fuels.
Moreover, with increasing global energy demands and the urgent need for alternatives to carbon-based sources, hydrogen emerges as a promising contender. The efficiency of energy conversion through fuel cells can result in lower operational costs and a more sustainable energy framework.
The significance of the output products of hydrogen combustion cannot be overstated. Not only does water vapor emerge as the primary byproduct, but the implications for heat generation and environmental impact must be carefully considered. By comprehensively analyzing these elements, the article aims to clarify the role of hydrogen fuel cells in the evolving energy landscape.
In this narrative, we will address the essential components and types of hydrogen fuel cells, elucidate the combustion process, and evaluate the products and their broader implications. Readers will gain a clear understanding of how hydrogen can be harnessed effectively, fostering a dialogue around its increasing integration into energy systems.
Fundamentals of Hydrogen Fuel Cells
Understanding the fundamentals of hydrogen fuel cells is essential for grasping their role in modern energy systems. Fuel cells convert chemical energy directly into electrical energy through electrochemical reactions. This process eliminates the need for combustion, leading to higher efficiency and fewer emissions, especially when compared to traditional power sources. As the global community seeks sustainable solutions to energy challenges, hydrogen fuel cells emerge as a pivotal technology, offering both versatility and environmental benefits.
Definition of Fuel Cells
Fuel cells are electrochemical devices that convert hydrogen and oxygen into electricity, water, and heat. By leveraging the distinct properties of these elements, fuel cells achieve a clean energy output. When hydrogen molecules react with oxygen at the anode in a fuel cell, the resulting electrochemical reaction separates the electrons, creating an electric current. Thus, the fundamental operation of fuel cells revolves around this simplistic yet efficient conversion of energy.
Components of Fuel Cells
Electrolyte
The electrolyte plays a crucial role in a hydrogen fuel cell by facilitating the transport of ions between the anode and cathode. Traditionally, this material is often a polymer or ceramic that allows protons to pass while blocking electrons. The key characteristic of an effective electrolyte is its conductivity. For example, the proton exchange membrane is particularly popular due to its lightweight properties and high conductivity at various temperatures.
A unique feature of the electrolyte is its ability to operate under different conditions, which can be advantageous for various applications. However, some electrolytes may have limitations related to durability and operating temperature range. This factor can impact overall performance and longevity of the fuel cell system.
Anode
The anode is the electrode at which oxidation occurs. In a hydrogen fuel cell, hydrogen gas enters the anode side, and the oxidation reaction splits hydrogen molecules into protons and electrons. A key characteristic of the anode is its surface area, which influences the rate of the reaction. Increasing the surface area enhances performance, making materials like platinum suitable for this application.
A unique feature of the anode is its ability to serve as both a conductor and a catalyst. While this dual function enhances performance, it also raises concerns regarding costs and resource availability. The use of precious metals can make hydrogen fuel cells expensive, requiring ongoing research into alternative materials.
Cathode
The cathode is paramount to the operation of a fuel cell, as it facilitates the reduction reaction. Oxygen from the air enters the cathode side, where it combines with the protons migrating through the electrolyte and the electrons returning from the external circuit. The key characteristic of the cathode is its capacity to manage the influx of oxygen and its reaction efficiency. Like anodes, cathodes must also maximize surface area to maintain optimal performance.
A unique feature of cathodes is that they can utilize various materials, providing flexibility in fuel cell design. However, challenges arise in terms of durability and degradation, which can influence operational stability over time, necessitating further research into improving material longevity.
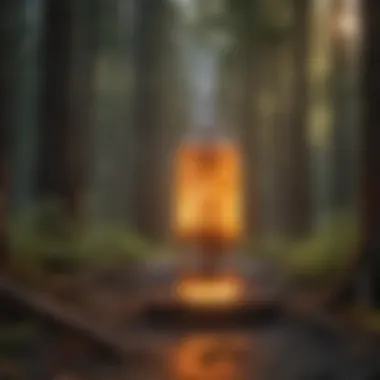
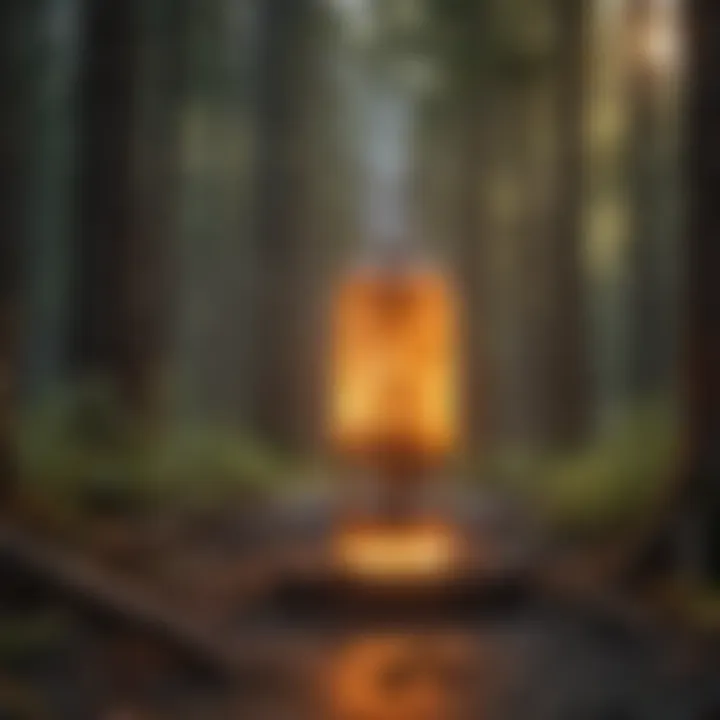
Types of Hydrogen Fuel Cells
Hydrogen fuel cells are categorized into several types, each suited to specific applications and requirements.
Proton Exchange Membrane Fuel Cells
Proton exchange membrane fuel cells (PEMFCs) are widely recognized for their applicability in transportation and portable power generation. They operate at relatively low temperatures, making them suitable for a variety of environments. A key characteristic of PEMFCs is their quick start-up time and high power density. This adaptability makes them a beneficial choice for applications like fuel cell vehicles.
A unique feature of PEMFCs is their need for pure hydrogen fuel, which can be a limitation in infrastructure development. Additionally, the expense of the membrane material can affect the overall cost of the system.
Phosphoric Acid Fuel Cells
Phosphoric acid fuel cells (PAFCs) are another type, typically used in stationary power generation. They operate at higher temperatures than PEMFCs and use phosphoric acid as the electrolyte. A key characteristic of PAFCs is their resilience to fuel impurities, making them a reliable choice for applications that may not have ultra-pure hydrogen available.
The unique feature of PAFCs is their ability to generate waste heat, which can be harnessed for co-generation applications. However, their lower power density compared to PEMFCs means they are not as suitable for mobile applications.
Solid Oxide Fuel Cells
Solid oxide fuel cells (SOFCs) represent a solid-state option suitable for high-temperature applications. They use a ceramic electrolyte and can operate on various fuels, including hydrogen and methane. A key characteristic of SOFCs is their high efficiency, especially in combined heat and power systems. This makes them a strong candidate for stationary applications in industrial facilities.
A unique feature of SOFCs is their flexibility in fuel choice; however, their requirement for high operational temperatures can lead to materials challenges and increased costs. This necessitates research into new materials and configurations to enhance performance and efficiency.
This exploration of the fundamentals of hydrogen fuel cells sets a foundation for understanding their combustion processes and the products resulting from those reactions.
The Combustion Process of Hydrogen
The combustion process of hydrogen is a critical element in the discussion of fuel cells and energy production. Understanding this process reveals insights into the efficiency and environmental impact of using hydrogen as a fuel source. Hydrogen combustion involves chemical reactions that are both fascinating and complex, marking a shift from traditional fossil fuels toward more sustainable energy solutions. The benefits, such as lower emissions and higher energy output, position hydrogen as an appealing option for various applications.
Chemical Reactions Involved
The basic chemical reaction for hydrogen combustion can be represented by the equation:
[ 2H_2 + O_2 \rightarrow 2H_2O + Energy ]
In this reaction, hydrogen gas reacts with oxygen to form water vapor, releasing energy in the process. This reaction is straightforward, yet it has profound implications for energy generation. The absence of carbon dioxide emissions further establishes hydrogen as a clean energy carrier compared to traditional fuels. Additionally, hydrogen's high energy content per unit mass makes it an effective fuel choice for applications that demand efficient energy delivery.
Energy Release Mechanism
The energy release mechanism during hydrogen combustion results primarily from the formation of water molecules. When hydrogen and oxygen combine, the bond-forming process releases energy that can be harnessed for work. This exothermic reaction is characterized by its rapid nature, leading to swift energy release, which is crucial in applications such as automotive fuel cells.
Unlike fossil fuels, the hydrogen combustion process generates significantly less heat impacting the environment negatively. Moreover, understanding this mechanism helps in optimizing fuel cell designs to maximize efficiency and longevity.
Comparison with Fossil Fuels
Comparing hydrogen combustion to fossil fuel combustion reveals stark contrasts. Fossil fuels like petroleum and natural gas release not only carbon dioxide but also other pollutants such as sulfur oxides and nitrogen oxides. These emissions contribute to environmental degradation and climate change. In contrast, hydrogen combustion primarily yields water vapor, substantially reducing the carbon footprint.
This comparison underscores the relevance of hydrogen as a future fuel source, promoting lower-impact energy strategies. Furthermore, hydrogen's ability to provide energy without the associated negative byproducts creates an interesting dialogue about the potential for a cleaner, more sustainable energy future.
"Hydrogen is the most abundant element in the universe, and unlocking its energy potential can revolutionize our approach to clean energy."
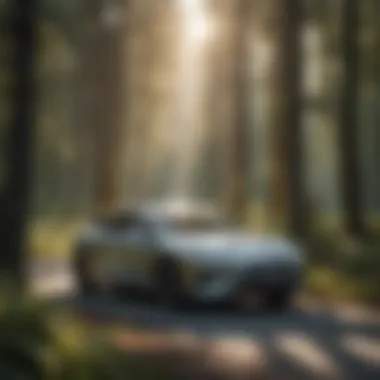
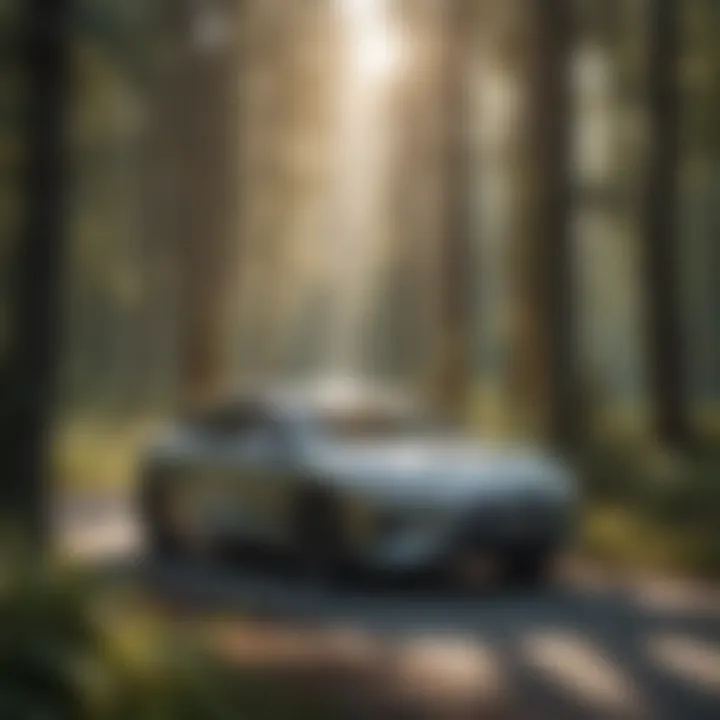
Overall, comprehending the combustion process of hydrogen is vital for tapping into its full potential as a sustainable energy resource. Recognizing the advantages it holds over fossil fuels positions hydrogen not merely as a fuel but as a pivotal player in the transition to greener energy solutions.
Output Products of Hydrogen Combustion
Understanding the output products of hydrogen combustion is essential for evaluating the viability of hydrogen fuel cells as an energy source. The effectiveness and overall impact of these systems largely depend on what byproducts are produced during the combustion process. The main products, which include water vapor and heat, reveal significant benefits and considerations associated with hydrogen usage in various applications.
Water Vapor as a Byproduct
When hydrogen combusts, one of the primary byproducts formed is water vapor. This occurs through the chemical reaction between hydrogen and oxygen where water is the result of these two elements combining. This outcome is noteworthy for several reasons:
- Environmental Friendliness: Unlike traditional fossil fuels that emit carbon dioxide (CO2) and other pollutants, the combustion of hydrogen produces only water vapor, contributing significantly to reducing greenhouse gases.
- Efficiency Indicator: The amount of water vapor can indicate the efficiency of the combustion process. Higher efficiency usually correlates with ideal combustion conditions, leading to higher water production.
- Humidity Concerns: Although water vapor is clean, it can be a concern in high concentrations, especially in enclosed spaces, where it can influence humidity levels. Managing these outputs requires careful consideration of operational environments.
Heat Generation
Combustion of hydrogen generates substantial heat, which has both positive and negative aspects. The generated heat is a direct output of the energy conversion process and is important for:
- Energy Utilization: The heat produced can be harnessed for various applications, including heating, electricity generation, and process energy in industrial settings. This utilization makes hydrogen a versatile fuel option.
- Engine Operation: In the context of fuel cells and other engines, this heat can help in the functioning of the system, ensuring optimal operations. However, it may also necessitate cooling solutions to avoid overheating.
- System Efficiency: Monitoring heat output can provide insights into the efficiency and effectiveness of the combustion system. Excessive heat may indicate inefficiencies requiring further investigation.
Impact on the Environment
The environmental impact of hydrogen combustion is a significant aspect of its appeal and ultimately its market adoption. Hydrogen's capability to produce clean energy has far-reaching implications:
- Reduction of Carbon Footprint: The main environmental benefit stems from the almost complete elimination of CO2 emissions during combustion, which aligns with global efforts to combat climate change.
- Air Quality Improvement: As pollutants from combustion of traditional fuels are minimized, air quality improves, benefiting public health and ecosystems.
- Sustainability Potential: Hydrogen produced from renewable resources can create a closed-loop system that enhances sustainability. However, it is crucial to ensure that the overall life cycle of hydrogen—from production through combustion—remains environmentally sound and renewable.
"The environmental benefits associated with hydrogen combustion signal a significant potential for its role in sustainable energy systems."
In summary, the output products of hydrogen combustion are critical components in assessing the overall value and impact of hydrogen fuel cells. By understanding the significance of water vapor, heat generation, and environmental implications, stakeholders can make informed decisions about the integration of hydrogen technologies into current and future energy systems.
Applications of Hydrogen Fuel Cells
Hydrogen fuel cells are increasingly gaining attention for various applications across multiple sectors. Their significance lies in their potential to provide clean energy, mitigate environmental concerns, and reduce dependence on fossil fuels. The versatility of hydrogen fuel cells allows them to be utilized prominently in transportation, stationary power generation, and industrial applications. Each of these contexts underscores different advantages and opportunities that hydrogen technology presents.
Transportation Sector
Fuel Cell Vehicles
Fuel cell vehicles (FCVs) exemplify a critical area of hydrogen fuel cell application. One specific aspect is their capacity to convert hydrogen gas into electricity through electrochemical reactions, which powers the vehicle. A key characteristic of FCVs is that they emit only water vapor as exhaust, making them an environmentally friendly alternative to traditional gasoline and diesel vehicles. This eco-friendliness is a major reason for the growing popularity of FCVs in the conversation about sustainable transportation.
One unique feature of FCVs is their relatively quick refueling time, comparable to conventional vehicles. This advantage is crucial for consumer acceptance. However, drawbacks exist, such as the currently limited fueling infrastructure and the cost of hydrogen production, which can hinder widespread adoption. Overall, FCVs contribute positively to the reduction of greenhouse gas emissions, aligning with global sustainability goals.
Hydrogen-Powered Buses
Hydrogen-powered buses represent another promising aspect of hydrogen fuel cell technology in the transportation sector. These buses utilize similar principles as FCVs and provide public transport systems with a cleaner alternative. A significant advantage of hydrogen-powered buses is their capability to support longer routes without requiring frequent refueling stops, which is vital for urban transit networks.
Their key characteristic is the larger fuel cell capacity compared to passenger vehicles, allowing for extended operational ranges. As cities strive for lower emissions, hydrogen-powered buses are becoming a favored choice for public transportation authorities. However, as with FCVs, challenges remain, particularly relating to infrastructure development for hydrogen refueling stations and the initial capital costs of deploying such technology. Nevertheless, they offer a compelling solution to city pollution and traffic congestion problems.
Stationary Power Generation
Stationary power generation using hydrogen fuel cells is another significant application. This method allows for the generation of electricity at a set location, such as residential buildings, commercial facilities, or industrial sites. A notable benefit is the possibility of providing uninterrupted power supply, especially in areas vulnerable to outages. Hydrogen fuel cells can operate continuously as long as hydrogen is supplied, making them reliable sources of energy.
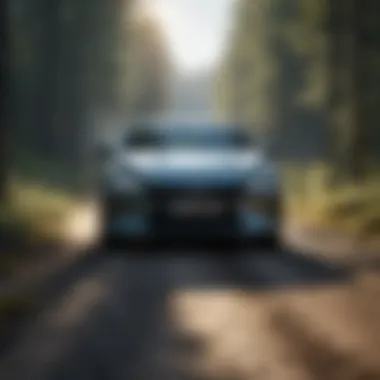
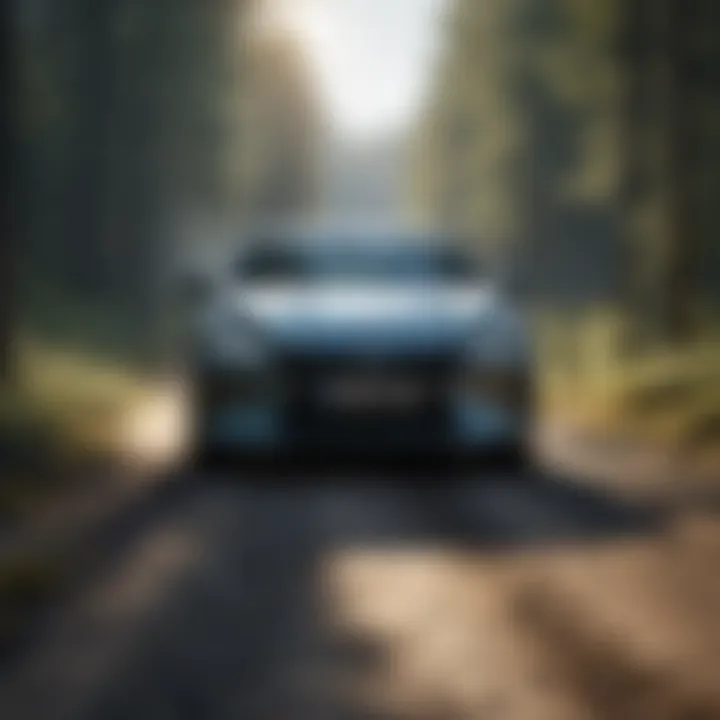
Furthermore, the transition to hydrogen for stationary power generation offers a reduction in harmful emissions and aligns well with renewable energy sources when hydrogen is generated from sustainable practices. Challenges in this area include the economic feasibility and efficiency of hydrogen production processes, which must improve to increase market competitiveness. Nonetheless, stationary hydrogen fuel cells illustrate the potential of hydrogen technology in diversifying energy supplies.
Industrial Applications
Energy Storage Solutions
In industrial settings, hydrogen fuel cells are particularly valuable as energy storage solutions. Hydrogen can be produced during periods of low energy demand or from surplus renewable energy. This stored hydrogen can later be utilized when energy demand peaks, acting as an effective buffer in energy systems.
One key characteristic of energy storage utilizing hydrogen is its long-term storage capability. Unlike batteries, hydrogen can be stored for extended periods without significant loss of energy. This feature enhances its attractiveness as a tool for stabilizing energy supply, especially in industries that rely on continuous, reliable power. However, the conversion efficiency from electricity to hydrogen and back can be lower than that of batteries, which remains a challenge for broader adoption.
Backup Power Systems
Backup power systems employing hydrogen fuel cells are another essential application in industrial contexts. As industries increasingly rely on uninterrupted power, the use of hydrogen fuel cells provides a viable solution in case of power failures. They offer a reliable and environmentally friendly alternative to traditional diesel generators.
The key characteristic is their ability to seamlessly integrate into existing power systems, providing backup without adding to emissions. One unique feature of these systems is their scalability, meaning they can be customized to fit the energy needs of various industrial applications. The initial investment and installation costs can be high, which might deter some organizations. Despite this, backup power systems utilizing hydrogen fuel cells are significant for enhancing energy resilience and sustainability in the industrial sector.
The Future of Hydrogen Fuel Technology
The significance of hydrogen fuel technology lies in its potential to revolutionize energy systems. As the world grapples with climate change and the depletion of fossil fuel resources, hydrogen presents a sustainable alternative. This energy carrier can be produced from renewable resources, offering a clean way to power various sectors. Its versatility in usage across transportation, electricity generation, and heating makes it crucial for future energy strategies. Understanding the future of hydrogen technology is vital as it shapes energy policies, market dynamics, and environmental sustainability.
Research and Development Trends
In the domain of hydrogen fuel technology, research and development are critical. Current trends include advancements in hydrogen production techniques, such as electrolysis and reforming processes using renewable energies. This evolution allows for a more efficient transition from fossil fuels to renewable sources. Increasing investment from both government and private sectors shows promise in advancing hydrogen fuel technologies. Moreover, innovations in fuel cells themselves, focusing on enhancing efficiency and longevity, are essential to make hydrogen a viable mainstream energy source.
Challenges and Limitations
Despite the potential of hydrogen as an energy carrier, challenges persist.
Cost of Hydrogen Production
The cost of hydrogen production remains one significant barrier. Currently, methods like electrolysis are expensive. The price is largely influenced by electricity costs, which can fluctuate greatly. Alternative production methods, such as steam methane reforming, involve higher carbon emissions, which are contrary to the goal of sustainability. Thus, while there is advancement, the economic feasibility remains a decisive factor for adoption. Reducing production costs through innovation is crucial for the future of hydrogen in energy markets.
Storage and Distribution Issues
Storage and distribution issues pose additional challenges. Hydrogen has a low energy density by volume, which complicates its transportation. It must be compressed or liquefied to be stored efficiently. This process incurs high energy use, further impacting economic viability. Pipelines and transportation infrastructure need substantial investments for safe hydrogen distribution. Addressing these storage and distribution complexities is essential for an effective hydrogen fuel framework.
Policy and Regulatory Considerations
Policy and regulatory considerations are pivotal for supporting hydrogen's integration into the energy system. Governments worldwide are beginning to formulate policies that incentivize hydrogen technology development. These regulations can drive funding towards research and development, support infrastructure projects, and establish safety standards. A well-structured policy framework can enhance investor confidence and market development for hydrogen technologies, setting the stage for a sustainable energy future.
"The transition to hydrogen fuel technology requires coordinated efforts among governments, industries, and research institutions. Collaboration is key to overcoming the hurdles that currently exist."
In essence, while hydrogen fuel technology faces various challenges, it also holds considerable promise. The future direction relies on continual innovative research, addressing cost barriers, tackling logistical issues, and fostering supportive policies. Stakeholders in the energy sector must stay informed about these critical developments to utilize hydrogen's full potential.
Ending
The conclusion of this article serves as a critical focal point, encapsulating the significance of hydrogen fuel cells within modern energy discourse. In recent years, hydrogen has emerged as a pivotal player in the search for sustainable energy solutions. Exploring the intricacies of combustion processes and the byproducts produced lays a foundation for understanding the broader implications of hydrogen utilization.
One of the key elements discussed throughout this article is the impact hydrogen fuel cells have on reducing greenhouse gas emissions. This is a notable benefit, especially in comparison to traditional fossil fuels. By emitting primarily water vapor when hydrogen is combusted, we can mitigate the detrimental air quality issues associated with combustion-based energy processes. Thus, acknowledging these environmental advantages is fundamental for stakeholders in energy sectors.
Next, this conclusion highlights the importance of ongoing research and development in fuel cell technology. Advancements in production methods and improvements in the efficiency of hydrogen fuel cells are imperative. Overcoming existing challenges, such as the high cost of hydrogen production and storage, remains crucial for the broader adoption of this technology.
Furthermore, it is essential to recognize the policy implications related to hydrogen fuel cells. Regulation and financial incentives can promote hydrogen as a viable alternative energy source, driving more research investments and commercial applications. Such measures can accelerate the transition to a more sustainable energy landscape, benefiting economies, environments, and societies alike.
In summary, the conclusion synthesizes the essential elements explored within this article, emphasizing that understanding hydrogen fuel cells goes beyond mere technical knowledge. It encompasses broader considerations for energy efficiency, sustainability, and policy frameworks. As such, this article provides a comprehensive overview for forestry professionals and academics, urging them to consider hydrogen's role in the future of clean energy.