Microsatellite Genotyping: Techniques and Applications
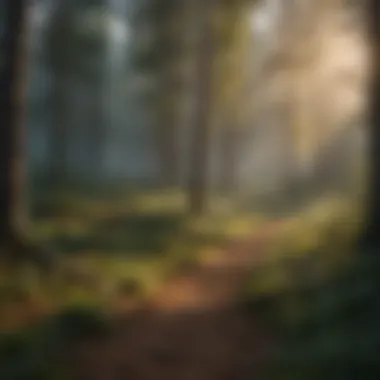
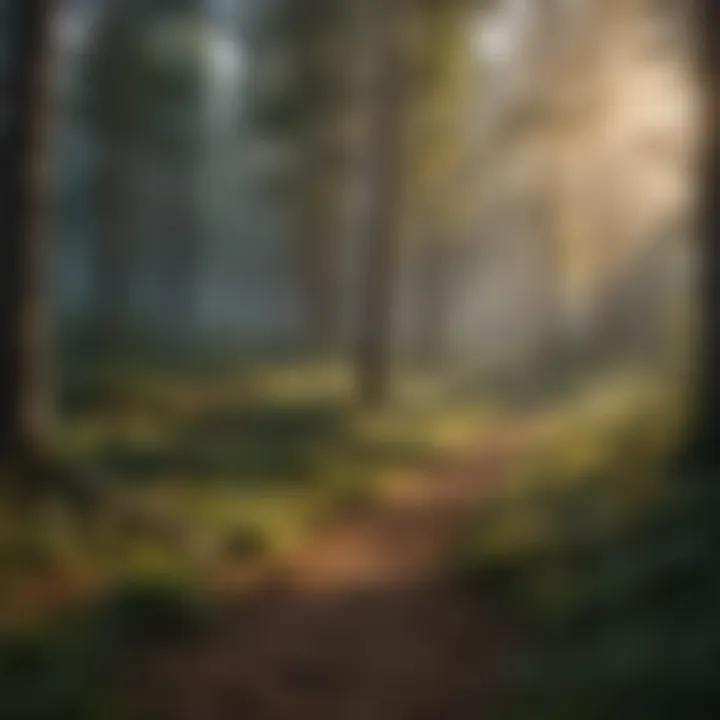
Intro
Microsatellite genotyping stands at the forefront of genetic research, presenting opportunities for in-depth analysis of genetic structures within species. By employing microsatellite markers, researchers dissect complex genetic traits and understand population dynamics. This article covers several aspects of microsatellite genotyping, including methodologies, applications, and implications for conservation and woodland management. By focusing on ecological aspects, we can better appreciate how this technique aids both scientific inquiry and practical conservation efforts.
Understanding Woodland Ecosystems
Importance of Biodiversity in Forests
Biodiversity is the foundation of resilient woodland ecosystems. Diverse species contribute to ecosystem stability, enhance productivity, and regulate services such as soil formation and nutrient cycling. A forestβs genetic diversity is particularly vital as it allows species to adapt to changing environmental conditions. This adaptability underpins the resilience of both flora and fauna against diseases and climate shifts.
The significance of biodiversity extends to human benefits, including medicinal resources, recreational opportunities, and aesthetically pleasing landscapes. Forests rich in biodiversity can also provide sustainable resources, vital for communities relying on natural ecosystems.
Role of Forests in Climate Regulation
Forests play a crucial role in climate regulation by acting as carbon sinks. Trees absorb carbon dioxide during photosynthesis, thereby reducing greenhouse gases in the atmosphere. This process is essential in mitigating climate change impacts. Additionally, forests contribute to local climate moderation, enhancing rainfall distribution and preventing soil erosion.
"The intricate relationships within woodland ecosystems underscore the importance of maintaining genetic diversity through methods like microsatellite genotyping."
Sustainable Forestry Practices
Principles of Sustainable Forestry
Sustainable forestry is essential for balancing ecological health with economic needs. Key principles include conserving biodiversity, maintaining ecosystem functions, and ensuring the regeneration of tree species. Sustainable practices promote selective logging over clear-cutting, allowing for habitat preservation and soil health maintenance. Furthermore, integrating local communities in the management process fosters stewardship and sustainable resource use.
Case Studies of Successful Implementations
Practical examples spotlight successful sustainable forestry practices. The Forest Stewardship Council certification offers a framework for responsible forest management, ensuring practices align with ecological and socio-economic standards. Similarly, the Reduced Impact Logging method minimizes damage to surrounding trees and ecosystems, enhancing regeneration potential.
Woodland Stewardship Techniques
Forest Management Plans
Effective management begins with well-structured plans. Forest management plans outline objectives, inventory resources, and define methods for sustainable practices. These documents guide actions toward conservation, ensuring alignment with long-term ecological goals. Key components involve periodic assessments and adaptive management strategies to address emerging challenges, from invasive species to climate change.
Conservation Strategies
Conservation strategies anchored in genetic research can enhance woodland stewardship. Microsatellite genotyping aids in understanding genetic diversity within populations, informing actions aimed at preserving species. For instance, identifying unique genetic populations helps prioritize areas for conservation efforts.
In summary, microsatellite genotyping serves as a powerful tool for genetic analysis within woodland ecosystems. Understanding this relationship contributes not only to scientific knowledge but also to the practical application of conservation strategies. As the field evolves, further advancements promise to deepen our understanding and enhance stewardship and management practices.
Understanding Microsatellites
Microsatellites, also known as simple sequence repeats (SSRs), are short, repetitive DNA sequences that play a crucial role in genetic studies. Understanding microsatellites is essential as they provide insights into genetic variability and population structure. Their unique properties enable researchers to utilize them in various fields such as ecology, conservation genetics, and evolutionary biology.
The importance of microsatellites lies in their abundance throughout the genome. They are widely distributed and their mutations can lead to significant genetic diversity. This makes them ideal for studying relationships among individuals and populations. For conservation efforts, microsatellites can be used to assess genetic health, manage breeding programs, and monitor the effects of habitat changes.
Definition and Characteristics
Microsatellites are composed of repeating units of 1 to 6 base pairs in length. Their polymorphic nature makes them valuable markers for genetic analysis. Each individual can have different numbers of these repeating units, which helps in distinguishing between them at a genetic level. The primary characteristics of microsatellites include their high mutation rate and ease of amplification via techniques like PCR. These traits contribute to their widespread utility in genetics.
Functions in Genomic Architecture
Microsatellites serve several functions within the genomic architecture. They can influence gene expression and function, contributing to phenotypic differences among individuals. Moreover, their presence in non-coding regions can play a role in genomic stability. Changes in the number of repeat units can lead to various genetic disorders. Thus, studying microsatellites helps in understanding both normal biological processes and pathological conditions.
Types of Microsatellites
There are several types of microsatellites based on the length of their repeat units. Each type has its unique aspects and applications.
Dinucleotide Repeats
Dinucleotide repeats comprise two base pairs that repeat in succession. They are often found in gene regions, making them useful for assessing population genetics. Their primary characteristic is having a relatively stable mutation rate compared to other types. This stability makes dinucleotide repeats a popular choice for applications requiring high-resolution genetic information. However, their limited variability in some species may restrict their usefulness in certain contexts.
Tetranucleotide Repeats
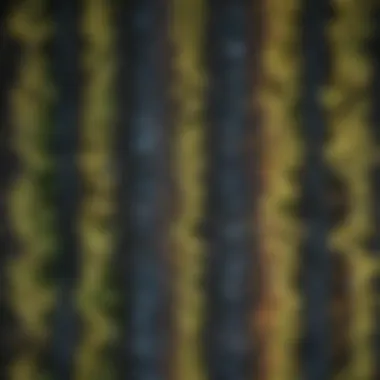
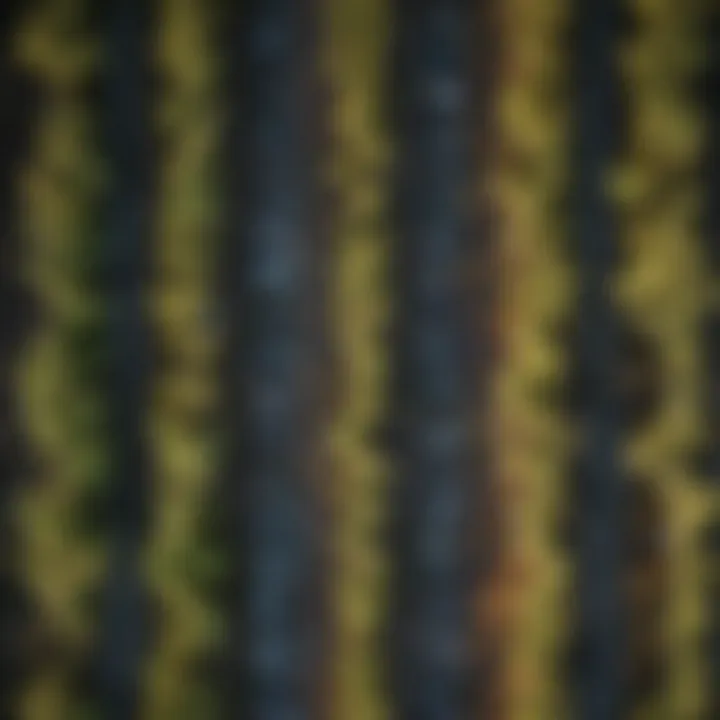
Tetranucleotide repeats are sequences where four base pairs are repeated. They tend to be more abundant and exhibit higher variability than dinucleotide repeats. This high variability is an advantage in population genetic studies, allowing for more precise and informative analyses. Tetranucleotide repeats are also less prone to mutations that can lead to genetic diseases, making them safer for study. Their frequent presence within coding regions further enhances their relevance to functional genetics.
Pentametric Repeats
Pentametric repeats consist of five base pairs that repeat. These are less common compared to the previous types but can provide distinct benefits. The key characteristic of pentametric repeats is their relatively high mutation rate, which can yield significant genetic information. While useful in certain contexts, their rarity can make it challenging to consistently find suitable markers for study. This aspect may limit their applications in broader population analyses.
Understanding and utilizing the specific types of microsatellites allows researchers to tailor their studies to obtain the most relevant genetic insights necessary for addressing their objectives.
The Science of Microsatellite Genotyping
Microsatellite genotyping serves as a critical tool in genetic research, offering insights into the genetic variation and population structure of organisms. This section outlines essential principles associated with microsatellite genotyping and highlights key techniques that enhance the analysis process. Understanding these facets is vital for forestry professionals and academics who seek to employ microsatellite markers for various applications, particularly in conservation, population biology, and ecosystem management.
Principles of Genotyping
Microsatellite genotyping is fundamentally rooted in the detection of short tandem repeats (STRs) in DNA sequences. These STRs can vary greatly among individuals, making them suitable for assessing genetic diversity and relatedness within populations.
The primary principle involves amplification of these regions using specific primers through techniques like Polymerase Chain Reaction (PCR). This amplification process allows researchers to produce enough DNA for accurate analysis. Along with amplification, the allelic variation at these loci is evaluated, providing insights into population dynamics.
In addition, enhanced resolution and reproducibility of genotyping are crucial in ensuring data reliability. This aids in minimizing errors associated with allelic dropout or misinterpretation of genotypes, primary concerns when conducting genetic studies.
Key Techniques in Genotyping
Genotyping utilizes various methodologies. Each has specific advantages that contribute to the overall goals of genetic research. Three predominant techniques include:
Polymerase Chain Reaction (PCR)
Polymerase Chain Reaction (PCR) is a fundamental technique in genotyping, providing a means to amplify specific DNA segments. PCR's contribution to microsatellite genotyping cannot be overstated, as it allows for the identification of polymorphic markers, even from limited DNA samples.
The key characteristic of PCR lies in its sensitivity. It can detect minute amounts of DNA, which is crucial when working with samples that may have degraded due to environmental conditions. This makes PCR a beneficial choice in the field of microsatellite genotyping.
A unique feature of PCR is its versatility, as it can be tailored to amplify various types of microsatellite loci. However, there are disadvantages such as potential for contamination or false positives, which require stringent controls and validation steps in the experimental design.
Fragment Analysis
Fragment analysis is another technique integral to microsatellite evaluation. It involves the separation and sizing of PCR products based on their length differences, which directly correlate to the number of repeats present.
The primary characteristic of fragment analysis is its ability to provide precise quantitative data regarding allele sizes. This accuracy makes it a popular choice for microsatellite genotyping, especially when elucidating population structure.
A notable advantage of fragment analysis is its efficiency in processing numerous samples simultaneously, which is crucial in large-scale ecological studies. However, it requires access to specialized equipment like capillary electrophoresis, which may limit its accessibility in some research settings.
Sequencing Approaches
Finally, sequencing approaches, including Sanger sequencing and next-generation sequencing, play a significant role in confirming microsatellite identities and analyzing their complexity. These techniques provide a high degree of accuracy, allowing for the determination of exact sequences at microsatellite loci.
The main characteristic of sequencing approaches is their capacity to provide comprehensive data about genetic variation at an unprecedented resolution. This makes them a highly valuable tool in research environments that demand in-depth genetic analysis.
Moreover, sequencing can unearth allelic variations not easily detected by other methods, thus broadening the understanding of genetic diversity. However, sequencing can also be costly and time-consuming, particularly when analyzing large datasets.
Overall, the science of microsatellite genotyping is marked by diverse approaches that enhance the understanding of genetic diversity and population structure. These techniques each have unique features and challenges, making them suitable for different research objectives. As a result, a deep comprehension of these methodologies allows for more effective applications in fields such as conservation biology and forest management.
Applications in Ecology and Conservation
Understanding the applications of microsatellite genotyping is essential for its role in ecology and conservation. Microsatellite markers provide valuable insights into genetic variation, structure, and differentiation within and among populations. These insights inform diverse fields, including conservation strategies, species management, and ecological studies. The benefits of applying microsatellite markers are profound, as they help unravel genetic relationships that can guide decisions in ecosystem management.
Population Structure Analysis
Microsatellite genotyping allows researchers to analyze the population structure of various species. By examining allele frequencies and distributions, scientists can identify genetic differentiation among populations. This differentiation is critical for understanding gene flow and migration patterns. Population structure analysis enhances our understanding of species adaptability to changing environments, especially in fragmented habitats. Variations observed in populations can indicate how well these populations might respond to environmental changes or anthropogenic impacts.
Genetic Diversity Assessment
Assessing genetic diversity through microsatellite markers is a vital component of conservation genetics. Higher genetic diversity typically correlates with increased resilience to diseases and environmental changes. Conversely, low genetic diversity can signify a population at risk of extinction. Microsatellites enable the measurement of genetic polymorphism, helping scientists monitor the health of populations over time. This assessment is particularly important for endangered species, where maintaining genetic diversity is crucial for their long-term survival and viability.
Conservation Genetics
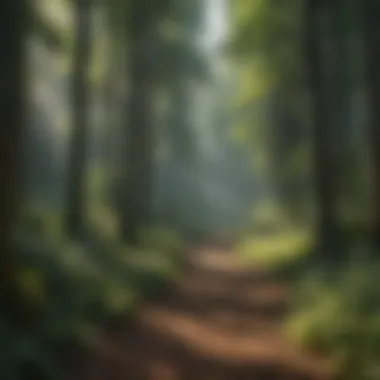
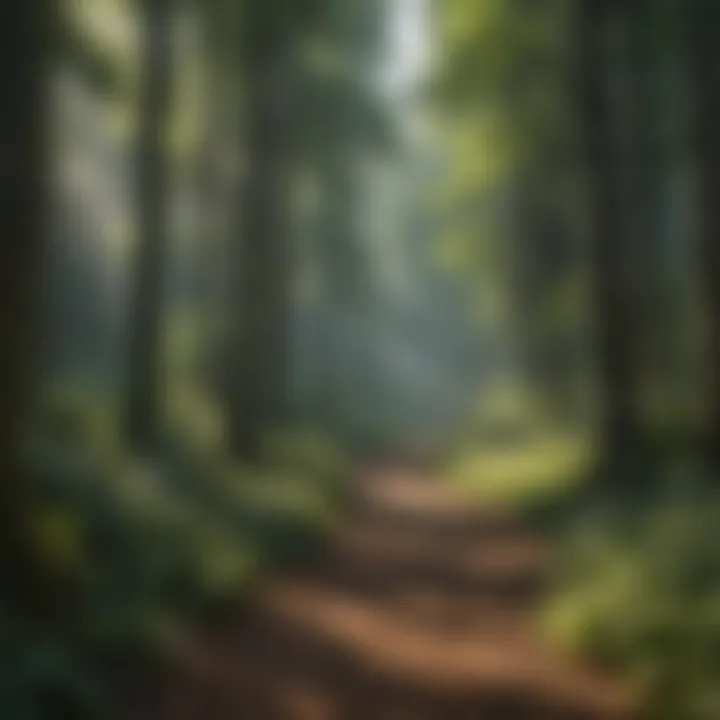
The field of conservation genetics directly benefits from microsatellite genotyping in several ways.
Endangered Species Management
In endangered species management, microsatellite analysis aids in the identification of specific genetic lineages. Understanding these lineages helps prioritize conservation efforts for distinct populations that may have unique adaptations. A key characteristic of this approach is its ability to provide insights into genetic drift and inbreeding levels. This information is highly beneficial for resource allocation in conservation programs. By focusing on genetic diversity, managers can make informed decisions about breeding strategies and habitat preservation. However, the challenge remains in collecting data from accessible ranges where endangered species live, leading to potential gaps in understanding their genetic status.
Restoration Ecology
Restoration ecology benefits from microsatellite genotyping by testing the genetic integrity of restored populations. The key characteristic of restoration ecology is its focus on restoring ecological functions and processes. Analyses involving microsatellites can inform which genetic stock to use in restoration activities. A unique feature of using microsatellites is their fine-scale resolution of genetic data, which is crucial for effective restoration planning. The advantages of employing this technique include improving the chances of success for restoration initiatives. However, limitations may arise when determining how much genetic variation is necessary to promote resilience in restored populations.
Implications for Forestry and Woodland Stewardship
The implications of microsatellite genotyping in forestry and woodland stewardship are profound. These molecular markers provide crucial data for understanding genetic variation within tree populations, which is essential for managing forests sustainably. By assessing genetic diversity, professionals can make informed decisions that aid in conservation and effective resource management. The ability to monitor genetic health and ecological dynamics positions microsatellite analysis as a pivotal tool in forestry.
Forest Health and Biodiversity
Microsatellite genotyping plays a key role in maintaining forest health and biodiversity. Genetic diversity within tree species is essential for resilience against diseases and environmental changes. Using microsatellite markers, researchers can identify genetic variation among populations. This helps prioritize conservation efforts where genetic diversity is low. Effective forest management relies on safeguarding not just individual species but the richness of genetic material that sustains ecosystems.
"Sustainable forestry practices hinge on understanding the genetic makeup of forest populations."
Moreover, maintaining biodiversity through genetic monitoring supports ecosystem services. Healthy forests with a variety of species can better support wildlife habitats, carbon sequestration, and soil health. Understanding the genetic landscape contributes directly to enhancing ecosystem resilience.
Monitoring Ecosystem Changes
Another crucial implication of microsatellite genotyping is its capacity for monitoring changes in ecosystems over time. As external pressures such as climate change and invasive species increase, the genetic monitoring of populations allows for early detection of shifts in biodiversity. By tracking genetic alterations, researchers can assess the impacts of environmental changes quickly and effectively. This intel helps in preparing adaptive management strategies to mitigate adverse effects.
In addition, microsatellite markers can help identify whether native species are being outcompeted or hybridized with invasive species. The outcome informs conservation strategies aimed at protecting native flora and fauna, which is vital for ecosystem stability.
Adaptive Management Strategies
Microsatellite genotyping supports adaptive management strategies in forestry. Adaptive management refers to a systematic approach to managing natural resources that integrates learning and knowledge. Insights gained from genetic data enable forest managers to adapt their practices based on observed genetic variations and responses to environmental stressors.
Implementing these findings fosters more resilient forest ecosystems. For instance, when certain tree varieties show greater survival rates in response to changing climates, forest managers can adjust planting strategies accordingly. This kind of flexibility is necessary for thriving ecosystems in a rapidly changing world.
Technological Advancements in Genotyping
Technological advancements have transformed the field of microsatellite genotyping, enhancing both the accuracy and efficiency of genetic analyses. Innovations such as next-generation sequencing and advanced bioinformatics tools have not only improved the quantity of data that can be processed but also the depth of insights that can be gleaned from such data. As the capabilities of these technologies expand, they offer new avenues for researchers to explore genetic diversity and population structure. Considerations related to cost, accessibility, and the necessary expertise for employing these techniques are crucial for forestry professionals and academics alike.
Next-Generation Sequencing
Next-generation sequencing (NGS) has revolutionized how microsatellite markers are analyzed. Compared to traditional methods, NGS provides a high-throughput approach, allowing multiple loci to be sequenced simultaneously. This capability significantly reduces the time and resources needed for genotyping.
Some benefits of using NGS in microsatellite analysis include:
- Increased Resolution: NGS can identify many more alleles at a given locus compared to conventional methods. This heightened resolution is vital for understanding population genetics.
- Cost-Effectiveness: Though initial costs might be high, the per-sample cost decreases with the ability to analyze numerous samples at once.
- Data Richness: NGS generates vast amounts of data, opening the door for comprehensive genetic studies, including SNP discovery and analysis of population structure.
Despite these advantages, NGS also presents challenges. Bioinformatics support is required to manage and interpret the substantial datasets produced. Thus, a skilled team is often necessary to ensure that researchers can effectively analyze microsatellite data generated by this technology.
Bioinformatics Tools
With the advent of genomic technologies, bioinformatics has emerged as a critical component in microsatellite genotyping. Specialized software and tools have been developed to help analyze complex datasets. These tools perform various tasks, from managing data to statistical analysis and visualization.
Key bioinformatics tools utilized in microsatellite genotyping include:
- Allele Calling Software: Programs like GeneMapper and STRand assist in identifying alleles from raw data generated by genotyping techniques. They improve accuracy in allele identification, essential for high-quality genetic analysis.
- Population Genetics Software: Applications such as Arlequin and STRUCTURE help analyze genetic variation and population structure among microsatellite data. These tools facilitate understanding of genetic relationships and evolutionary patterns within and across species.
- Data Visualization Tools: Websites and software used for visualizing microsatellite data enhance understanding of complex genetic information. Visualization can highlight trends and patterns in genetic diversity, aiding communication of findings among researchers and stakeholders.
The integration of bioinformatics tools with genotyping technologies enables researchers to handle the complexity of genetic data effectively, leading to more informed decisions in conservation and management strategies.
In summary, technological advancements, particularly next-generation sequencing and bioinformatics tools, are reshaping microsatellite genotyping. These innovations not only streamline the research process but also allow for deeper investigations into genetic diversity and population dynamics.
Challenges in Microsatellite Genotyping
Microsatellite genotyping provides valuable insights into genetic diversity and population structure. However, it also comes with a unique set of challenges that researchers and practitioners must navigate. Understanding these challenges is crucial as it impacts the accuracy of data obtained, decisions made in conservation efforts, and research outcomes. Evaluating these challenges allows for better strategies and solutions in enhancing microsatellite analysis effectiveness.
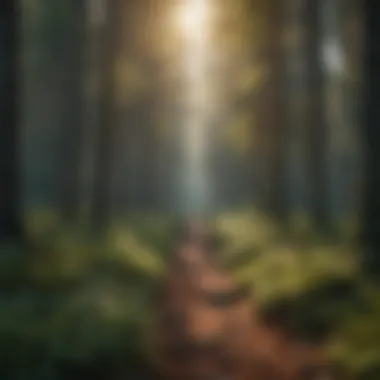
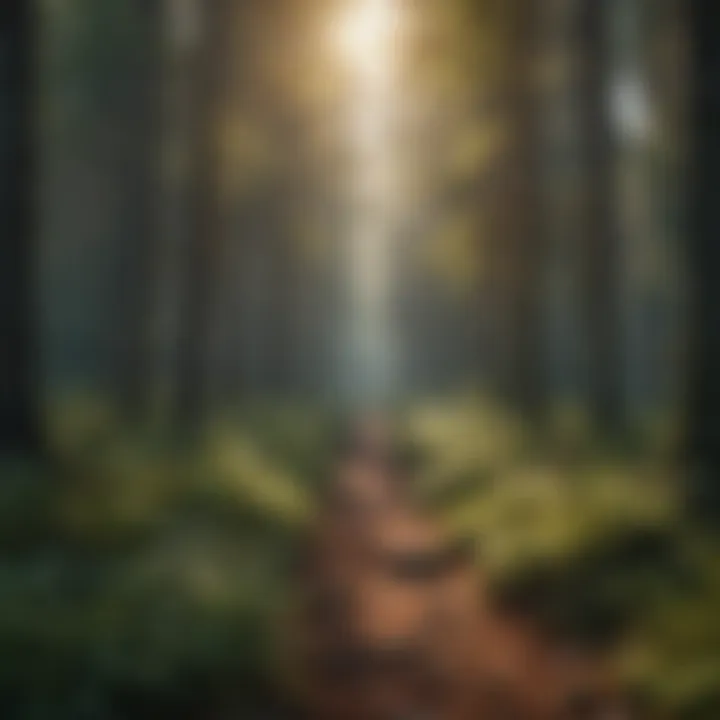
Allelic Dropout
Allelic dropout refers to the phenomenon where one allele of a heterozygous individual fails to amplify during the PCR process, leading to misleading genotyping results. This can happen due to several factors such as poor DNA quality, competition between alleles for amplification, or primer design issues. In practice, allelic dropout can significantly affect the inferred genetic diversity estimates, which are critical for conservation genetics. If allele dropout occurs frequently, it may result in an underestimation of heterozygosity and misinterpretation of population structure. Understanding this issue is important, as it can skew conservation strategies based on faulty genetic data.
To mitigate the effects of allelic dropout, researchers can implement specific measures, including:
- Ensuring high-quality DNA extraction techniques.
- Optimizing the conditions for PCR amplification.
- Using carefully designed primers that reduce competition.
- Utilizing multiplex PCR to increase the likelihood of obtaining both alleles without dropout.
Data Interpretation and Analysis
Data interpretation in microsatellite genotyping is another significant challenge. The complexity of genetic data requires proper statistical methods and bioinformatics tools for accurate analysis. Often, researchers may encounter issues related to the distinction between true biological patterns and artefacts introduced during the genotyping process. Misinterpretation can lead to incorrect conclusions regarding population health or genetic diversity.
To ensure robust data interpretation, one must consider several key factors:
- Statistical Power: Ensure that the sample size is sufficient to detect meaningful genetic variations.
- Model Selection: Choosing the correct statistical model for analyzing the data is crucial. Different models can yield different insights.
- Software Tools: Using reliable software, such as STRUCTURE or GenAlEx, is vital for proper analysis.
- Collaboration with Statisticians: Engaging experts in data analysis can greatly enhance the understanding of the results.
Future Directions in Research
The field of microsatellite genotyping is on the brink of significant evolution. Researchers and practitioners are increasingly recognizing the potential of this technique to address pressing challenges. As the understanding of the genetic underpinnings of species increases, it becomes paramount to integrate microsatellite analysis with other genomic tools. This integration enhances the depth of genetic data available, leading to more comprehensible insights in various fields including conservation and biodiversity.
Integration with Other Genomic Tools
Integrating microsatellite genotyping with next-generation sequencing (NGS) is a key focus for advancing research. Next-generation sequencing allows researchers to obtain a vast amount of data at a much faster rate and with reduced costs compared to traditional methods. By using NGS, scientists can simultaneously analyze microsatellites as well as genome-wide information, resulting in a comprehensive understanding of genetic variations.
The combined approach offers several benefits:
- Increased accuracy in genotype determination.
- Greater resolution in identifying population structures.
- Enhanced ability to detect allelic variation across populations.
- Improved assessment of genetic diversity.
Furthermore, bioinformatics tools play an essential role in analyzing the complex data generated through these integrations. They facilitate the interpretation of genetic data, allowing researchers to visualize relationships and genetic trends in greater detail.
Applications in Climate Change Studies
Another promising direction for microsatellite genotyping lies within climate change studies. Understanding how species respond to climate shifts is crucial for conservation efforts. Microsatellite markers can unravel the adaptive capacities of populations, shedding light on their resilience to changing environments. For instance, studies employing microsatellite analysis have found variations in genetic diversity correlated with climate variabilities.
This information can be instrumental in:
- Predicting species survival in shifting habitats.
- Identifying potential refugia for vulnerable species.
- Monitoring evolutionary responses to rapid environmental changes.
Ongoing research is pivotal. It helps inform management practices aimed at protecting biodiversity in the face of climate shifts. Integrating microsatellite genotyping in climate studies can significantly influence conservation strategies, ensuring that they are both effective and responsive to ecological changes.
Closure
In this article, we have explored the intricate world of microsatellite genotyping, elucidating its techniques, applications, and implications. The conclusion serves as a critical recap of why microsatellite genotyping is essential not just in the field of genetics but also in ecological and conservation efforts.
Microsatellites, or simple sequence repeats, play a pivotal role in understanding genetic variation within and between populations. Their high polymorphism makes them invaluable for assessing genetic diversity, which is especially beneficial in conservation genetics. By pinpointing variations, researchers and conservationists can develop targeted strategies for endangered species management and habitat restoration.
Furthermore, the significance of technological advancements in microsatellite research cannot be overstated. Emerging methods, coupled with traditional ones, provide a more comprehensive toolkit for researchers. These innovations enhance data accuracy and improve the reliability of genetic analyses in various ecological contexts.
Additionally, as climate change presents new challenges, microsatellite genotyping allows for detailed studies of how species adapt to shifting conditions. This understanding is crucial for developing effective conservation strategies and informing policy decisions regarding natural resources.
In summary, microsatellite genotyping stands as a cornerstone of genetic research and conservation efforts, bridging the gap between molecular biology and practical applications in forestry and ecology. Its continued relevance in these fields points to the necessity of ongoing research and integration with other genomic tools to face emerging environmental challenges.
Importance of References
Including a comprehensive list of references enhances the article's authority. It allows readers to verify claims, follow where data originated, and explore additional studies that may supplement their understanding. This transparency fosters trust and enhances the reader's engagement with the content. Reliable sources in scientific writing are paramount. This is especially true in a field as dynamic as genetics where methodologies evolve rapidly.
Specific Elements to Consider
When referencing works in the field of microsatellite genotyping, consider the following elements:
- Peer-Reviewed Journals: Articles published in journals such as Molecular Ecology or Conservation Genetics provide validation through community scrutiny.
- Books and Texts: Textbooks that focus on molecular biology or genetics give foundational context. For example, works that explain the principles of microsatellite dynamics can be particularly useful.
- Theses and Dissertations: Research originating from academic submission often presents detailed methodologies and innovative applications that are sometimes overlooked in established literature.
- Online Resources and Databases: Websites like Wikipedia or Britannica can help introduce concepts but should be complemented with primary research sources.
Benefits of Comprehensive Referencing
- Supports Claims: Each reference serves as a pillar supporting the arguments made in the article.
- Guides Future Research: Providing links to important studies encourages readers to build on existing knowledge.
- Facilitates Networking: References assist in connecting with other researchers or institutions involved in similar work.
- Enhances Learning Opportunities: Readers can gain access to diverse perspectives and methodologies relevant to their interests in genetics and conservation biology.
"Proper citation is not merely a formality; it is an ethical responsibility that nurtures academic integrity".
Using a clear, consistent referencing style, whether APA, MLA, or another format, ensures that citations are easy to follow and locate.
In summary, the references section plays a vital role in substantiating the information presented. By carefully selecting and accurately citing sources, the article can stand strong in the scholarly community, benefitting forestry professionals and academics alike.